Combined Heat and Power (CHP) plants are unsung microgrid heroes. Properly configured and designed CHP solutions can provide microgrids with resilient, low-carbon power. In fact, CHP remains a uniquely practical resource, especially for mission-critical facilities operating microgrids, because it can produce a continuous, controllable baseload source of electric and thermal energy.
Yet despite its incredible benefits, CHP’s potential may be limited as all-electric heating systems become more cost-effective. In the age of rapid decarbonization, campus leaders are turning to alternate district heating system technologies such as ground-sourced heat pumps or heat recovery systems, which—unlike CHP—would operate without direct input from fossil fuels.
CHP systems must adapt to a zero-emission future, or they risk becoming expensive, stranded assets.
Efficient, Reliable, Resilient Energy
An advanced microgrid integrates dispatchable generation, typically natural-gas-fired generators, creating an independent network of energy assets. Flexible energy resources enable the integration of intermittent, inverter-based energy sources such as solar photovoltaics (PV) coupled with battery energy storage solutions (BESS). Together, these distributed energy resources provide stable, resilient energy.
Santa Fe Community College’s 366-acre “educational microgrid” features a 12.5-kW solar tracker and 100-kW BESS. The college is currently planning for a campus-scale microgrid capable of indefinite microgrid operations. Plans include a 1.5-MegaWatt (MW) photovoltaic solar array, 1-MW, 770-kWH BESS that utilizes excess solar capacity, and—most importantly—a flexible 1-MW natural gas-fired generator.
Microgrids must match generation sources in real-time to the connected facilities’ load. Campus facility leaders have limited options if microgrid generation is based solely on intermittent resources. Very large batteries would need to account for inter-day load swings, with even more capacity necessary to account for extended and unplanned outages. These backup batteries would sit idle, charging in the event of an outage, providing little economic return.
Enter the anchor CHP system.
A CHP system provides a flexible, dispatchable electrical source that can respond in real-time to these system dynamics. CHP offers the added benefit of being highly efficient. Also known as “cogeneration,” CHP systems generate electrical power while capturing thermal energy that would otherwise be wasted. The captured heat is applied to on-site loads, creating a highly efficient, reliable, and resilient district energy system.
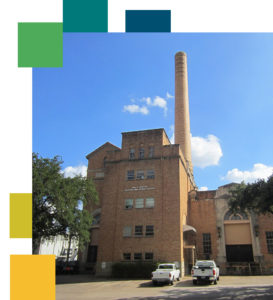
The University of Texas at Austin CHP power plant, originally built in 1929, can now generate 135 MW of peak power and 1.2 million lb./hr. of steam heating. Plans are underway to integrate on-campus solar photovoltaics.
One of the largest and oldest examples of an advanced CHP-based microgrid is at the University of Texas at Austin (UT). The UT Combined Heating and Power Complex provides 100 percent electricity and heating for 150 buildings across campus.
Through careful planning and thoughtful investment, the UT CHP microgrid is flexible enough to respond independently to changes in electrical and thermal loads while boasting one of the most efficient CHP systems in the world. The plant, originally built in 1929, can now generate 135 MW of power (62 MW peak load) and 1.2Mlb./hr. of steam heating.
CHP systems keep humming within a microgrid—even when solar PV production is low or batteries are depleted. Outside of planned maintenance activities, CHP plants provide uninterrupted yet efficient energy. CHP truly becomes the steady pulse for resilient and efficient microgrids.
Carbon Conundrums
Motivated by carbon-reduction mandates or stakeholder-driven sustainability commitments, campus decision-makers are increasingly choosing to shift to 100 percent renewables. In the not-distant future, several district energy systems will be capable of running entirely on zero-emission thermal sources.
Phasing out natural gas-fired systems—CHP included—may seem like an intuitive decarbonization measure. However, electrification will dramatically increase electrical demand, heightening the challenge for microgrid operations.
Greater reliance on the utility grid does not necessarily absolve campus leaders of their carbon footprint. Even if all-electric heating systems lower on-site (Scope 1) emissions, carbon accounting must consider the power grid’s mix of energy sources. In regions where the grid continues to rely upon fossil fuels, the power supply’s carbon footprint (Scope 2 emissions) may lead to greater overall emissions for an all-electric campus, particularly compared to an on-site CHP scenario. Notably, the Department of Energy indicates that a typical CHP system averages 700 pounds of carbon dioxide equivalent (CO2e) per MWh. In contrast, the current yearly average of purchased electricity within the PJM power grid territory averages 811 CO2e/MWh.
Compared to current operations, most campuses would experience significant pollution-reduction benefits upon transitioning to a CHP microgrid. The grid remains roughly 60 percent reliant on fossil fuels, while three-quarters of thermal energy comes from natural gas or heavy oil. A 1-MW CHP plant operating a natural gas-fired CHP would emit nearly half the annual carbon emissions of traditional district energy applications—largely due to the plant’s capture and reuse of thermal energy.
Facility leaders should consider how CHP systems offer other undeniable benefits, particularly at campus-scale, including:
- CHP systems are scalable and can be customized to meet a microgrid’s specific design requirements.
- CHP plants are far more energy efficient than traditional energy options, achieving a significant reduction in energy consumption and associated cost avoidance.
- Centralized CHP systems enable district energy campuses to provide heating and cooling services in a central location. This reduces the need for on-site equipment in campus buildings, freeing up valuable real estate for other core mission applications.
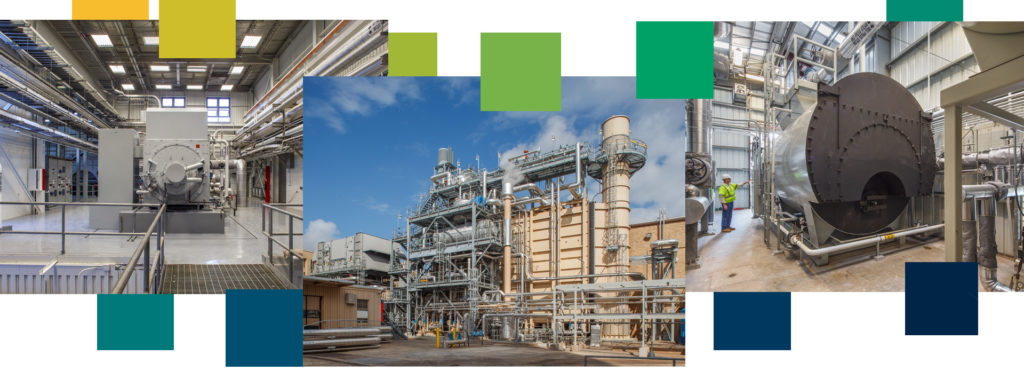
Texas A&M University is another prime example of thoughtful application of CHP as an anchor resource for a research intensive campus.
Alternative Fueling Options
Campus leaders may not have to choose between installing CHP and decarbonizing. Modern advances in combustion and emissions control are opening up possibilities for CHP systems to operate reliably on alternative, low-carbon fuel sources.
However, of the 215 currently operating CHP microgrids, only five rely entirely on renewable fuels, according to the U.S. Department of Energy. In practice, biofuel and renewable gas options remain expensive. Production and distribution can be unpredictable. Many facilities lack capacity for on-site fuel storage, a key requirement for resilient operations in a low-carbon environment.
CHP installations can operate using fuel cells—a reliable, efficient, and scalable source of electricity. Fuel cells generate electricity by reacting fuel with oxygen through an electrochemical process to generate electricity (and waste heat). The CHP system can generate zero-emission electricity if the fuel cells’ energy source is hydrogen, produced using renewables.
Conventionally, hydrogen is created from methane-based fuel sources—mostly natural gas or biogas. This can be relatively emission-intensive. Hydrogen production from unabated natural gas has an emissions intensity in the range of 10–14 tons of carbon dioxide equivalent per ton of hydrogen (CO2e/H2). Natural gas production’s upstream and midstream emissions are responsible for another one to five tons of CO2e/H2.
Hydrogen-powered fuel cells, turbines, and boilers may become cost-effective as fuel costs fall and distribution networks expand. Federally funded “hydrogen hubs” promise to increase “green hydrogen” supplies made with renewable electricity. Production currently remains limited, however, with much development pending.
Several universities are already exploring how to introduce green hydrogen into their energy mix. In 2021, Clemson University announced the H2-Orange pilot project in partnership with Duke Energy and Siemens Energy, incorporating green hydrogen as a low-carbon fuel source for the campus’ CHP plant. When the CHP system was installed in 2020, Clemson could decommission coal-fired boilers, reducing carbon emissions by 49,000 metric tons annually. At scale, the H2-Orange project will serve as another step in Clemson’s transition to carbon neutrality. Green hydrogen can lower emissions associated with the plant’s 60,000 lb./hr. of thermal energy production and 17.8 MW of electricity generation.
Don’t Sleep on the Benefits of a Thoughtful CHP Application
District energy facility leaders can either wait until cost-effective, low-carbon hydrogen resources become widely available—still several years out—or act now, installing a thoughtful CHP system with immediate economic and environmental benefits.
A properly configured and designed CHP solution can provide resilient microgrid power with dramatically reduced carbon intensity. Practical facility leaders are installing CHP systems with future-proofing in mind, designing provisions that accommodate alternative fueling sources as they become viable.
If a campus or district shares the goal of resilience with decarbonization, an optimized CHP solution presents facility leaders with a large step change and serious “bang for the buck.” CHP remains a powerful solution for obtaining every useful bit of energy from the system’s fuel sources.
Perhaps most importantly, CHP plants make microgrids work. All-electric heating systems increase reliance on limited power supplies, while CHP plants improve district energy systems’ resilience. Electric heating systems place added strain onto microgrids, while CHP plants enhance microgrid reliability.
CHP remains a solution few can afford to ignore.
Roger Copeland, PE, is the power and utilities practice lead at Burns Engineering. He can be reached at [email protected].